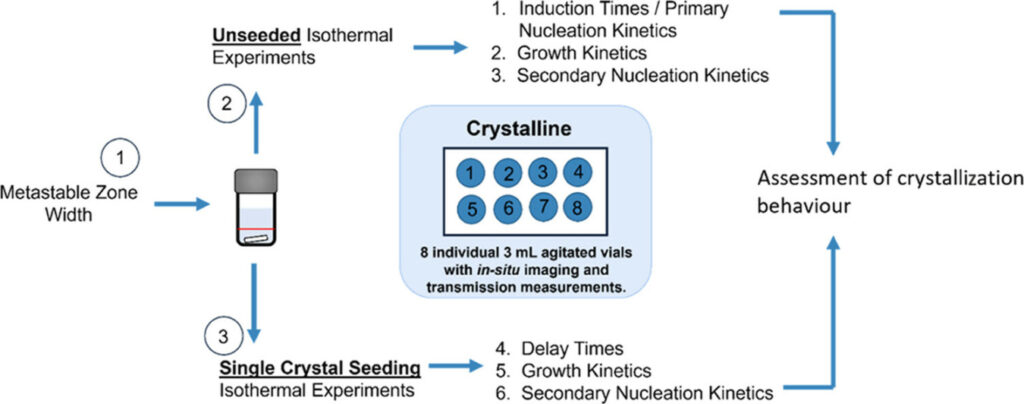
In the world of material science, the ability to accurately determine the crystalline structure of materials is paramount. Crystallinity, a property that significantly impacts a material’s physical and chemical behavior, plays a crucial role in industries ranging from pharmaceuticals to advanced materials engineering. Precision in detecting crystallinity is not only a scientific necessity but also a regulatory demand in many sectors. This article delves into cutting-edge approaches to crystallinity analysis, highlighting advancements that are shaping the future of material characterization.
The Importance of Crystallinity in Material Properties
Crystallinity is a fundamental property that defines how atoms or molecules are arranged in a material. Highly crystalline materials exhibit ordered structures, which often translate into properties like greater strength, stability, and thermal resistance. Conversely, amorphous materials, with their disordered structures, may offer enhanced flexibility or solubility.
For industries like pharmaceuticals, detecting crystallinity is critical because it can directly influence drug efficacy and stability. Similarly, in polymers, the degree of crystallinity determines characteristics like tensile strength, durability, and thermal behavior. As a result, accurate and reliable methods for analyzing crystallinity are indispensable for quality control and research.
Traditional Methods for Crystallinity Analysis
Several established techniques are widely used to assess crystallinity in materials:
- X-ray Diffraction (XRD):
XRD is one of the most popular methods for identifying crystalline structures. By analyzing the diffraction patterns created when X-rays interact with a sample, scientists can determine the material’s crystalline and amorphous phases. - Differential Scanning Calorimetry (DSC):
DSC measures the heat flow associated with phase transitions in a material, such as melting or crystallization. The enthalpy changes observed during these transitions provide insights into the degree of crystallinity. - Fourier Transform Infrared Spectroscopy (FTIR):
FTIR identifies vibrational modes in molecular structures, offering indirect information about crystallinity through the analysis of specific functional group frequencies.
While these techniques are reliable, they often have limitations in resolution, sensitivity, or sample preparation requirements. Advancements in crystallinity detection method development have sought to overcome these challenges, enabling more precise and efficient analyses.
Advances in Crystallinity Detection Methods
Modern advancements in crystallinity detection have introduced novel techniques and enhanced existing ones, allowing for greater accuracy, efficiency, and versatility. Key developments include:
1. Micro X-ray Diffraction
This technique combines the high resolution of traditional XRD with the ability to analyze extremely small samples or localized regions within a material. It is particularly useful for heterogeneous samples or materials with complex structures.
2. Raman Spectroscopy
Raman spectroscopy is gaining traction as a complementary tool for crystallinity analysis. It provides molecular-level insights and can differentiate between crystalline and amorphous regions with high spatial resolution. Its non-destructive nature makes it ideal for delicate samples.
3. Electron Diffraction
For nanomaterials and microcrystals, electron diffraction offers unparalleled resolution. The shorter wavelength of electrons compared to X-rays enables detailed structural analysis, even for samples that are too small for conventional XRD techniques.
4. Machine Learning Integration
Artificial intelligence is transforming crystallinity detection by improving the interpretation of complex datasets. Machine learning algorithms can identify patterns and anomalies in diffraction data, making analyses faster and more accurate.
Applications of Advanced Crystallinity Analysis
The ability to accurately detect crystallinity is invaluable across various fields:
- Pharmaceuticals: Understanding the crystalline structure of active pharmaceutical ingredients (APIs) is critical for drug formulation, stability studies, and regulatory compliance. Enhanced detection methods allow researchers to optimize drug efficacy and shelf life.
- Polymers and Composites: In materials science, crystallinity analysis informs the development of polymers with desired mechanical and thermal properties, aiding in the creation of lightweight, durable materials.
- Energy Storage: In the energy sector, the crystallinity of battery materials like cathodes and anodes influences performance metrics such as capacity and cycling stability. Advanced techniques help optimize these materials for better energy storage solutions.
- Nanotechnology: High-resolution crystallinity analysis is essential for characterizing nanomaterials, whose properties often depend on subtle structural details at the atomic level.
Overcoming Challenges in Crystallinity Detection
Despite significant progress, challenges remain in achieving consistently accurate and reproducible crystallinity measurements. For instance, preparing samples without altering their structure can be difficult, particularly for soft or temperature-sensitive materials. Additionally, interpreting data from heterogeneous samples requires expertise and advanced computational tools.
To address these issues, researchers are focusing on developing standardized protocols, improving instrumentation sensitivity, and integrating complementary techniques to provide a comprehensive picture of material properties.
The Future of Crystallinity Detection
The evolution of crystallinity detection is poised to continue, driven by the demands of modern industries and the availability of innovative technologies. Future developments may include:
- Real-time Monitoring: Portable instruments capable of in-situ crystallinity analysis could enable real-time monitoring in manufacturing environments.
- Multimodal Techniques: Combining techniques like XRD, Raman spectroscopy, and electron diffraction in a single workflow could offer unparalleled insights into material properties.
- Enhanced Computational Tools: Advances in machine learning and AI are expected to streamline data analysis, making high-resolution techniques accessible to more laboratories.
Conclusion
Crystallinity detection is a vital aspect of material characterization, influencing everything from pharmaceutical development to cutting-edge materials science. Advances in detection techniques have significantly improved the ability to analyze crystalline structures, providing researchers and industries with tools to develop innovative products and meet rigorous quality standards.
As technology continues to evolve, the precision, efficiency, and accessibility of crystallinity detection methods will only improve, empowering scientists to explore new frontiers in material science. Whether in drug development, energy storage, or nanotechnology, these advancements will play a crucial role in shaping the future of research and innovation.